August 30, 2023
By
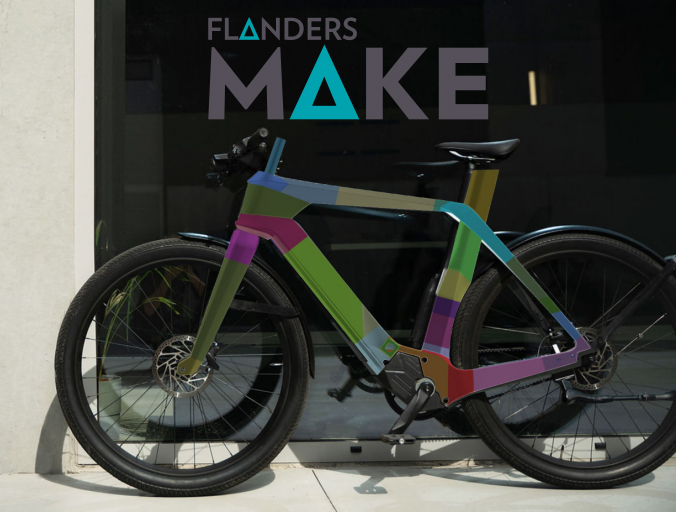
The demand for lightweight solutions in our products is growing rapidly. Reducing the weight of structures helps us in many ways, from enabling cyclists to ride faster to helping vehicles save fuel. However, mass-producing these lightweight structures has always been a challenge, balancing the need to save weight with the need for sufficient structural integrity to withstand all expected loads. Recent developments are further enabling and democratising these lightweight solutions on a larger scale. This is particularly the case with fibre-reinforced composites, where manufacturing processes can now be dramatically shortened for mass production and engineering calculations are becoming very precise. In a recent case study, we’ve worked with a number of partners to further improve these carbon fibre structures for the electric bicycle from Belgian bike manufacturer Specter.
The RightWeight InterReg project has been a catalyst for innovative SMEs in the North West Europe region to develop, prove and showcase their lightweight solutions. 4RealSim (NL) are experts in advanced structural calculations and offer a state-of-the-art solution for the optimisation of complex composite laminates, originally developed for the aerospace industry. Flanders Make has applied this method to a case study for the electric bicycle by Specter (BE), for which the composite structure was manufactured by CarbonMade (IT).
We find that the laminate optimisation method available in Collier Aerospace’s HyperX software can significantly reduce the time it takes to design complex composite structures, with added confidence in the functional performance of the resulting parts. Inherent in the method is a large amount of domain knowledge about composite design and manufacturing processes which is made available to the user in a natural and seemingly automated workflow. This makes it very easy to start from a simple FE model and arrive at an advanced and detailed laminate design, ready for production.
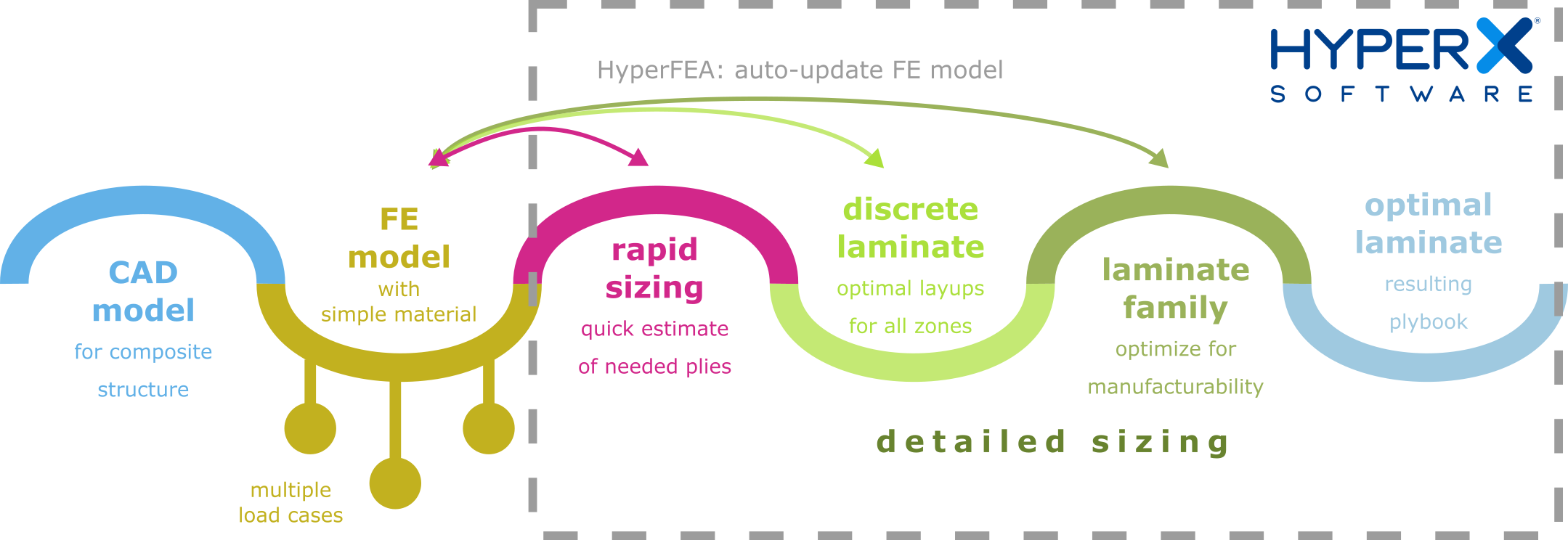
ADVANCED COMPOSITE PRODUCTION
Advanced composites are a class of fibre reinforced plastics (FRPs) with the best mechanical properties tailored to the application. The fibres, usually glass or carbon, are oriented to provide stiffness and strength where needed according to the load path. Different layers of continuous unidirectional (UD) or woven fibres can be stacked in different orientations as a laminate to achieve highly specialised mechanical properties.
The fibres are encapsulated in a matrix, which can be a thermoset resin, such as epoxy, or a thermoplastic polymer. A thermoset resin can be infused and cured for low volume production, while thermoplastic composites are more attractive for high-volume production due to faster production cycles, but also require heavier tooling.
Composite bicycle frames typically use prepreg (= pre-impregnated, but uncured) layers of carbon fibre with an epoxy matrix. These layers are then cut to size and stacked in the correct orientation in a mould. For complex shapes and load cases, certain zones will require more layers and different fibre orientations than others. Particular attention must be paid to continuity in the transition areas to avoid local stress concentrations that can lead to early damage and failure in service.
SPECTER E-BIKE: FRAME DESIGN AND REQUIREMENTS
The composite parts of the Specter e-bike are the frame, fork and handlebar. For our study of the optimal laminate, we focus on the frame and fork, which are subjected to the most critical load cases. These are three fatigue load cases (shown below) for which the frame and fork must withstand at least 10,000 cycles without notable damage or change in mechanical behaviour.
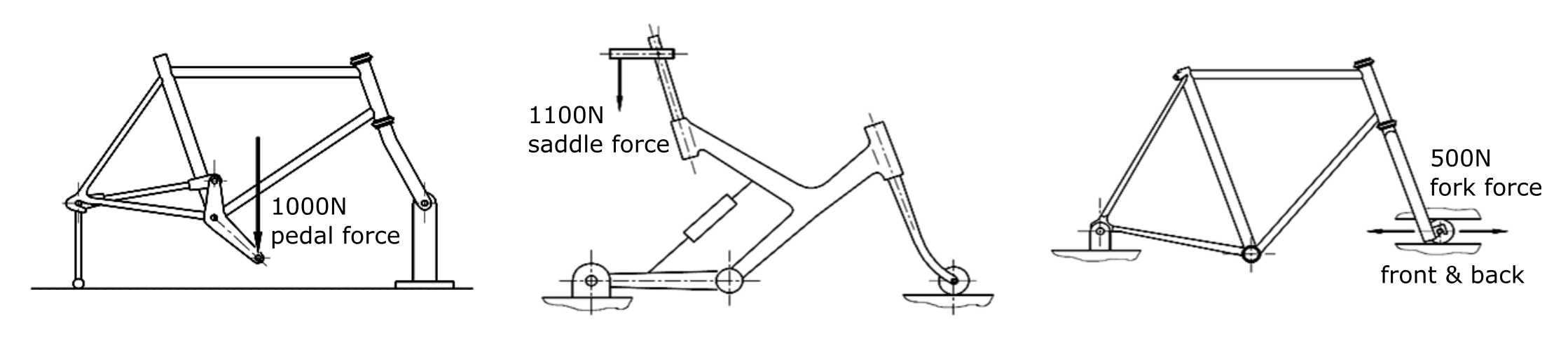
These load cases are first modelled using separate finite element software to evaluate the mechanical performance of the design. As we will be using the HyperX software for the subsequent laminate optimisation, we can simply assign uniform material properties for the FE model, e.g. 1mm aluminium, to the whole structure. This saves us the time of defining a laminate for each zone in the model.
HYPERX LAMINATE OPTIMISATION
The FE model and initial results are imported into the optimisation software. We can now select the material(s) to be used for the carbon e-bike. We choose a common – but high quality – carbon-epoxy UD tape with a layer thickness of 0.14 mm and a stiffness modulus in the fibre direction of 138 GPa.
The optimisation is performed for all load cases simultaneously. However, as composite fatigue is different from metal fatigue and depends on many factors, there is not (yet) a single, reliable model for calculating fatigue life. Therefore, it is common practice to translate a composite fatigue requirement into a static load case with a safety margin, in this case a safety factor of 1.5 is used.
1. RAPID SIZING OPTIMISATION
As a first approximation for switching to composite, the HyperX software takes the local force at each element of the mesh for all load cases considered and calculates the required number of composite layers in each specified zone, taking into account constraints on the number of plies in each direction.
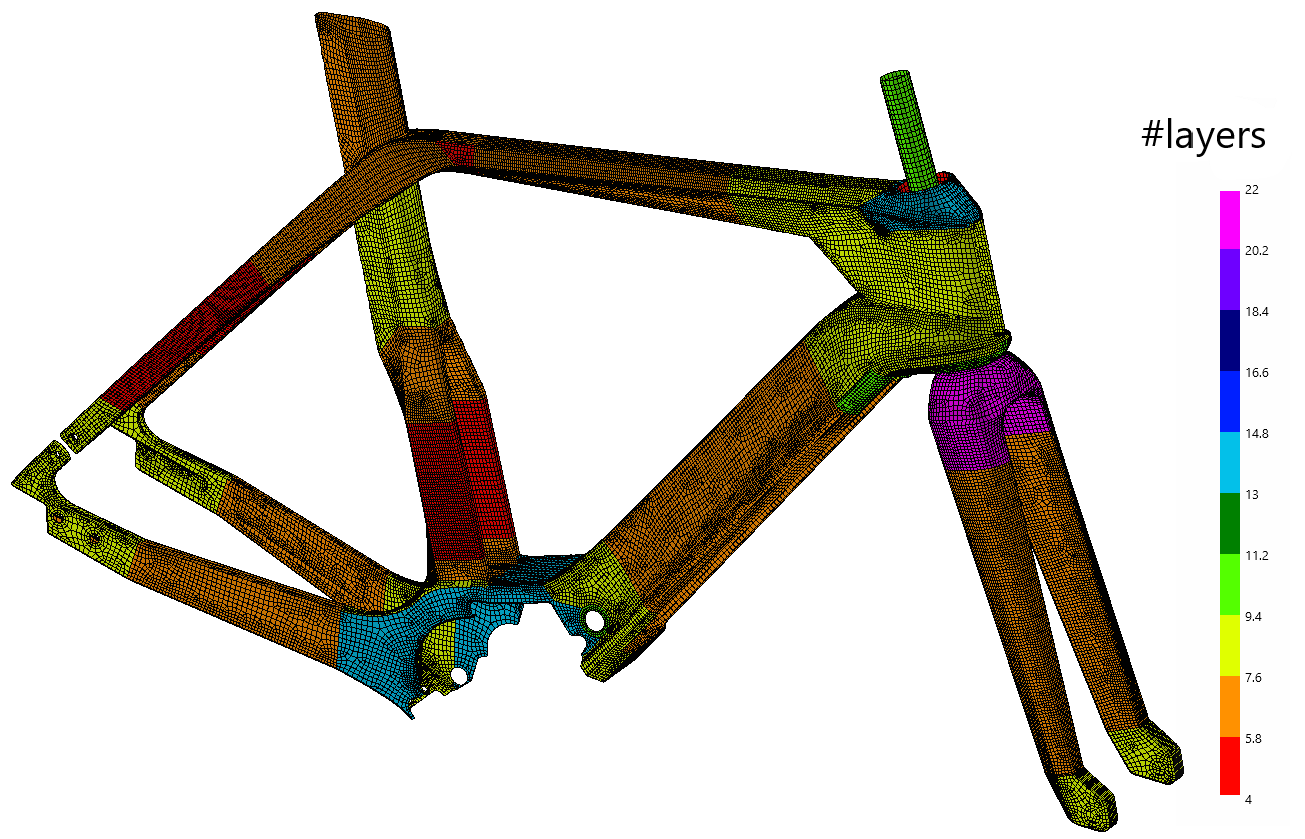
However, because the initial FE results were calculated for isotropic material, the load paths are no longer the same when orthotropic material is used and an update of the FE model is required. The assignment of composite laminates is automatically updated for each zone and the FE model is solved again for each load case (by coupling HyperX with the FE solver). Running the rapid sizing again already gives a good idea of how much composite material will be needed in each zone and what the final weight might be (1.4 kg).
2. DETAILED SIZING OPTIMISATION: DISCRETE LAMINATES
We have specified several constraints on what the final laminate should look like: minimum and maximum percentage of fibres in 0°, 45° and 90° orientations; symmetric and balanced layup; and continuous ply constraints across zones. The next step is to calculate what the optimal percentage is for each orientation, resulting in the effective laminates for each zone. Again, the FE model is updated and the sizing optimisation iterated.
From the resulting amount of layers in each orientation, the set of discrete laminates for all zones is generated, taking into account continuity across different zones. After verification with the FE model, these are the resulting laminate layups for the frame and fork, and the final weight is known: 1.5 kg!
3. DETAILED SIZING OPTIMISATION: MANUFACTURABILITY
The final step is to analyse how best to make the transition from one zone of thicker laminate to another zone with fewer layers so that the discontinuity is kept to a minimum and ply drop-offs do not lead to premature delamination. As a result, we know which layers should be continuous across zones and which should to be cut.
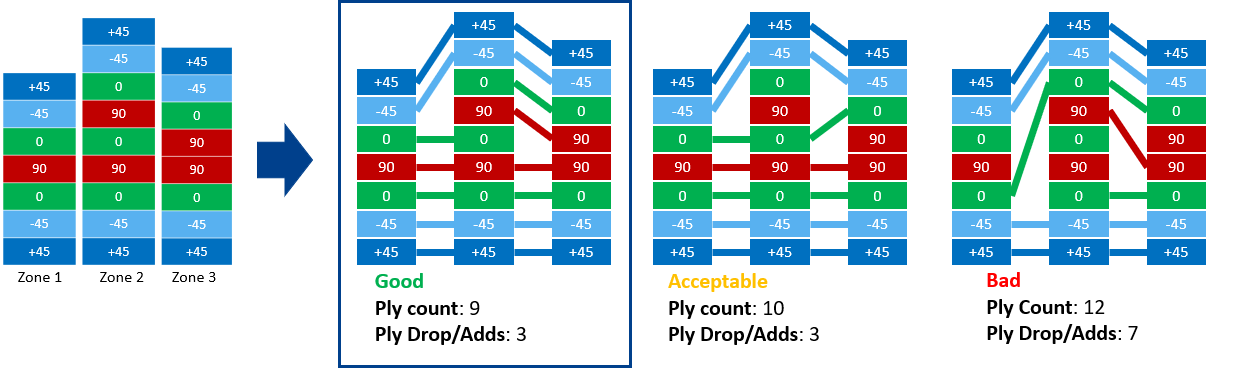
This information is used to create the plybook. This is the complete description of how each composite layer should be cut to size and where it should be placed in the mould and on top of the other layers. In other words, all the information needed to start manufacturing the parts for the e-bike!
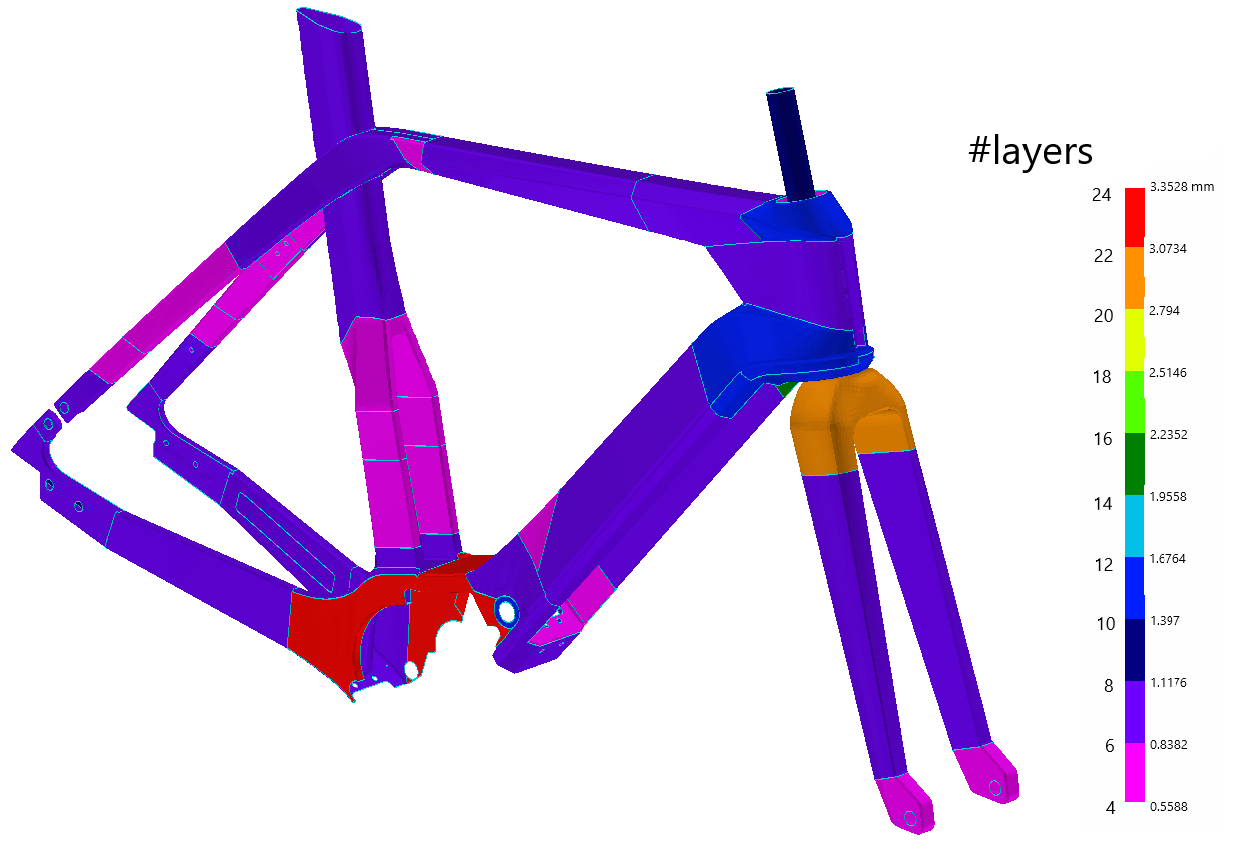
CONCLUSION
The final weight of 1.5 kg represents a weight saving of around 1/3rd for the composite parts of the existing Specter e-bike, which is a great achievement. These kinds of savings are very helpful for bike manufacturers in saving resources and making a faster and more dynamic bike. But the technique can offer a lot more in other fields, like making cars and other vehicles more fuel efficient.
The main conclusion, however, is that the laminate optimisation method available in HyperX (developed by Collier Aerospace) can significantly reduce the time taken to design complex composite structures compared to the traditional, iterative design workflow, which is often based on rules of thumb. The software contains a wealth of domain knowledge on composite design and manufacturability, made available through a modern user interface with a natural workflow. Starting from an FE model that has been quickly created with simplified material models, the software helps create a detailed laminate design that is ready for production in relatively short time (< 1 day) using the expert knowledge embedded in the tool. This idea also fits well with the way we create our industry-oriented research tools at Flanders Make.